In this Report
- Summary
- Introduction
- Assessment & Strategic Plans of Research & Activities
- Outcome Assessment
- Conclusions
You may also download the Energy 2100 Report in PDF format.
Energy 2100 Steering Committee Members
- Bruce E. Logan, Civil & Environmental Engineering (chair)
- Charles Anderson, Biology
- Rachel Brennan, Civil & Environmental Engineering
- Chris Giebink, Electrical Engineering
- Caitlin Grady, Rock Ethics Institute and Civil & Environmental Engineering
- Chris Rahn, Mechanical Engineering
- Susan Stewart, Aerospace Engineering
Acknowledgments
Support for the preparation of this report was provided through Penn State University’s Strategic Initiatives Seed Grants. We thank the following people at Penn State in the Institutes of Energy & the Environment for their help in preparing the final version of this report: Tom Richard, Director; Kevin Sliman, Communications Manager; Brenna Buck, Graphic Designer; and Tori Indivero, Writer/Editor.
Penn State is an equal opportunity, affirmative action employer, and is committed to providing employment opportunities to all qualified
applicants without regard to race, color, religion, age, sex, sexual orientation, gender identity, national origin, disability or protected veteran status. This publication is available in alternative media on request. U.Ed. RES 20-11.
Photo Credits: Patrick Mansell, Penn State; Kevin Sliman, Penn State; Adobe Stock
Summary
The global energy infrastructure is undergoing rapid change, with solar panel capacity doubling worldwide ever y two to three years, and wind energy now providing 7% of electrical power in the United States. As the national energy portfolio is becoming more diverse and complex, it will increasingly rely upon renewable power sources and complementary energy storage technologies. To provide avision for research, education, and outreach in renewable energy and associated activities, Penn State funded a study under the University Strategic Initiatives Seed Grant, in the area of “Stewarding Our Planet’s Resources.” This report provides a summary of activities at Penn State in renewable energy capture, conversion, and storage, and makes recommendations for strategic investment to enhance activities in renewable energy with the goal of further growing leadership and impact in energy activities based on carbon-neutral or carbon-reducing energy capture, storage, and utilization.
Key recommendations are:
- Building strength in solar and photonic energy research that emphasizes materials development and education
- Reinforcing wind energy activities in education and outreach and bolstering fundamental wind research
- Growing additional strength in electrochemistry research as a fundamental discipline related to these technologies
- Melding advances in wind and solar conversions with electrical energy storage through technologies such as batteries and hydrogen gas
- Invigorating activities in bioenergy with complementary activities in biomaterials
- Engaging businesses, communities, and governments in the transformation of our energy systems and infrastructure to a carbon-neutral and then carbon-negative economy
- Educating a workforce capable of integrating these technologies into our home, industries, and global economies
There are already exemplary activities at Penn State that need to be better communicated to our alumni and the public, but additional investment is needed to support these and to spark new renewable energy research, so that these activities will continue to grow
and become more impactful on the Pennsylvania economy in the coming years.
Introduction
Pennsylvania has always been at the forefront in the development of new energy sources, starting with extracting energy from wood, coal, oil, uranium, and most recently natural gas. The next evolution in energy conversion is already underway globally, with the cost of producing electricity from wind and solar power now equal to or less than that of natural gas in many locations. The unprecedented and rapid growth of solar panel production, which is currently doubling approximately every two years, could drive costs of solar electricity even lower, forever changing the landscape of our electrical power system. Wind power has also surged in recent years, making up 7% of the electricity generation in the US, with additional plans for increasing electricity production from wind by using off-shore energy systems. Hydroelectricity is the largest global source of renewable electricity, accounting for 17% of the world’s total electricity, with an estimated annual global growth of 3.1%. New energy conversion technologies are also being developed to capture energy from diverse sources, including biomass, low temperature and waste heat, and salinity gradients (between freshwater and seawater).
Penn State is well-known for its leadership in energy-related research, but the impressive research being conducted at Penn State in the area of renewable energy has not received sufficient national and global recognition. This quiet status of excellence in renewable energy research needs to change, through better communication and outreach, and it needs to grow by increased investments in these energy and associated technologies, as well as relevant areas of extension, policy, law, systems modeling, and economics, to further develop and integrate these technologies into our energy systems.
An increased emphasis on renewable energy research, education, demonstration, and outreach is important to both our aspiration to serve as America’s Energy University and for implementation of the Stewarding Our Planet’s Resources theme of Penn State’s Strategic Plan. Continued global growth in the use of fossil fuels is driving the unprecedented release of CO2 and other greenhouse gases, such as methane, into the atmosphere. In order to curtail and eventually achieve net negative greenhouse gas emissions, and in the absence of scalable approaches to carbon sequestration, fossil fuel use for energy production must be replaced by renewable and affordable sources of energy. Fortunately, there has been a substantial decline in the costs of producing electricity using renewable energy sources, which now provides economic incentives to switch from fossil fuels in many locations. However, further reductions in capital and operating costs for these renewable technologies, methods to reduce or eliminate the use of precious metals in these systems, and new energy technologies are needed to more rapidly transform an existing energy infrastructure that is heavily dependent on fossil fuels.
While renewable energy is the focus of Energy 2100, it is not the sole solution to climate challenges. The needed reduction of CO2 emissions will also require increased efficiencies in energy use and over 80 other solutions as outlined by Project Drawdown, many of which are active areas of research by hundreds of faculty, staff, and students. These reductions in greenhouse gas emissions can be achieved through conservation methods, particularly by designing and operating buildings to be more energy efficient, and by altering agricultural production methods, reducing food waste, and addressing a wide range of sustainable development goals.
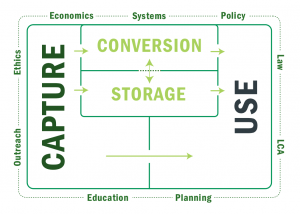
Penn State is already working on initiatives to reduce energy consumption in buildings, and it will host the first Drawdown International Conference in the fall of 2019. While conservation and other approaches are needed to reduce CO2 emissions, these subjects are not addressed further here.
Instead, this report focuses on renewable energy technologies and associated energy storage systems, rather than energy conservation or other changes needed beyond energy for drawdown of CO2.
Renewable energy systems will require an integrated approach to energy infrastructure that is responsive to changing spatial and temporal relationships between supply and demand. The intermittent nature of many renewable energy conversion technologies, the cyclical use of energy consumption, and the electrification of automobiles and other forms of transportation, will spawn an unprecedented need for massive energy storage systems. At present, 90% of renewable energy storage is achieved simply by pumping water uphill, and reclaiming the energy when the water flows back down using energy efficient turbines. While batteries already play an important role in conditioning electricity and stabilizing the grid from fluctuations on the scale of seconds to minutes, current costs are excessive for the hours and days of storage needed for an energy system dominated by solar and wind. Additional inexpensive methods for energy storage must be developed. For example, new forms of heat storage at very high temperatures could enable new methods of energy storage in the form of heat “batteries.” Unconventional batteries such as flow batteries, which store energy externally, could also impact larger scale energy storage. Although traditional batteries that have internal energy storage will remain a critical component of our energy infrastructure, there is a growing need for larger-scale, efficient, and more economical methods to store energy in order to balance electricity production with its use.
Technological solutions alone will not drive changes in our energy infrastructure. New technologies or changes in energy use can produce disruptive changes in our lifestyles, economic markets, and community structures. Wind farms, for example, may make sense from the perspective of technical energy production, but the sight of mountains covered with windmills may not be accepted even by the communities that they serve, and thus wind power might not be deployed even in technically sensible locations. Neighborhoods transformed to have all rooftops covered in solar panels could create conflict by clashing with the aesthetic values of a community. Energy systems of any type have potential environmental impacts which must be evaluated and mitigated as projects are developed. Renewables are no exception, so their development requires careful assessment of impacts of these technologies on the environment, including watersheds, avian species, and wildlife, as well as on human health and safety. Many renewable technologies also can require large capital investments. Therefore, even when changes in energy consumption might make economic sense in the long term, initial capital investments may not be possible for many people and communities with limited financial resources. Thus, any analysis of changes in energy systems will need to consider social and community values, which can vary on scales of neighborhoods, communities, counties, and states, as well as alternative financing strategies and the policy and governance mechanisms needed to address energy access and affordability as well as to support needed change.
Scientific discoveries, innovation in energy systems, and technology development and deployment will require collaborations among different groups of researchers. For example, chemical engineers producing hydrogen for use in fuel cells, synthesis of ammonia for fertilizers, or in oil refineries to produce liquid fuels such as gasoline, will need to work with electrical engineers and materials researchers if they are to change production methods to sustainably produce hydrogen from water using solar or wind energy. The location of hydrogen production could produce new challenges in transportation via pipelines or tanks. Harvesting additional energy from new environmental sources, for example from salinity gradients between rivers and oceans or dams, could similarly require new collaborations between engineers and politicians. For example, civil engineers can re-design both small and large dams currently being used solely for flood control to operate as new sources for local electricity production. However, there are legal issues that can prevent the dual use of dams for these purposes, and thus engineers will need to work with politicians, social scientists, and environmentalists to produce meaningful changes in the current water control systems. Increased collaborations among all these groups as well as with meteorologists and climate scientists will be needed to achieve greater resiliency of our water, transportation, and building infrastructures.
Changes in our energy infrastructure will require altering educational curricula and practices. Aside from direct technology development, education and research must include greater involvement of new disciplines and materials. For example, the strong growth of electron-based energy systems will require research into:
- How living systems carry out electron transfer during photosynthesis and other process.
- How electrons and CO2 can be used as starting materials for industrial processes.
- How automobiles are designed, maintained, operated, and driven.
- How our homes operate, and when and how electrical power is consumed on a daily or annual basis.
Fundamentals of electrochemistry and electrochemical engineering will need greater infusion into the modern scientific and applied science curricula. Various types of membranes and separators, which are used in batteries, fuel cells, chemical separation processes, and many new methods of energy storage and conversion, will need to be advanced in terms of achieving greater efficiencies, stabilities, and lifetimes. Smart systems that will link up micro- and large-scale power grids will need to be developed for our homes, infrastructure, and industries.
In 2018, Penn State provided seed grant funding for the project “Energy 2100: Ensuring a carbon-neutral, global energy economy” under the university’s Strategic Initiatives Seed Grant, in the topical area of “Stewarding Our Planet’s Resources.” The goal of that project is to drive additional research, innovation, and education in technologies that could be used for providing energy, in the form of transportable fuels and electricity, in an environmentally sound manner in order to use our planet’s resources in a sustainable manner. The year 2100 represents both the end of the century, as well as perhaps the end of coastal cities as they exist today due to a rise in sea level of as much as 2 to 4 meters (6 to 12 feet), not including storm surges. The vision for this initiative was to enable a future where renewable energy sources are readily available and at a cost comparable or less than existing fossil fuels, and a global economy that operates without releasing excess CO2 and other greenhouse gases into the atmosphere. Through that seed grant, the steering committee has worked with faculty in the summer and fall of 2018 to develop a series of strategic plans on different topics related to renewable energy research, education, and outreach.
These assembled plans (which can be found on energy2100.psu.edu) were then used to prepare this report. In the following sections of this report, the information in these plans has been synthesized and used to highlight important future directions for strategic investment that will improve the impact of the university’s activities in renewable energy, to enable Penn State to maintain its leading role in energy research as we evolve from a fossil infrastructure to an electron-based and carbon-neutral energy future.
Assessment & Strategic Plans of Research & Activities
Energy Conversion Technologies
Solar Energy
In the coming years and decades, solar-based energy technologies are likely the single most important component to the University’s renewable energy research and education portfolio. Penn State researchers have strength in thin film photovoltaic materials development, visibility to the local community through demonstrations projects such as the MorningStar Solar Home, and faculty and staff offer courses that can lead to a graduate certificate in Solar Energy as well as a Master of Professional Studies in Renewable Energy and Sustainable Systems (RESS) with a focus in Solar Energy. The solar energy community at Penn State, however, is a fragile and fragmented group that needs strong investment and support to produce a more stable, impactful, and cohesive team. Erosion and losses of the core of researchers in this area could greatly impede future research efforts and addition of faculty in this area. It is therefore imperative to develop additional leadership in solar research at both the component and system level through support of existing faculty as well as recruitment of new faculty to both science and engineering colleges. Faculty who are leaving should also quickly be replaced, and additional faculty lines are needed in the areas of photovoltaic materials and photonic energy research, particularly at the intersection with electrochemical fuels and feedstock. Attracting exceptional researchers in these areas can be achieved by leveraging the success and prominence of the Materials Research Institute (MRI) at Penn State. This flagship institute can help to draw in faculty who need world class materials and instruments to support their research.
Wind Energy
There is an extensive community of faculty and researchers at Penn State with experience or interest in various aspects of wind energy education and research. Given the right opportunities and incentives, internationally recognized faculty with expertise in various aspects of rotor and blade design for aircraft and rotorcraft can translate their expertise into research on wind energy blade design. There is justified optimism of new research and educational opportunities continuing in the future in wind energy systems, with 90 GW of wind power already in the US, another nearly 40 GW under construction, and over 100,000 people employed in this area. Research areas include topics associated with operation of wind turbines in difficult environments, such as in offshore areas or in situations relevant to possible blade icing, integration of wind-produced electricity into micro- and large-scale electricity grids, and science-based communication of educators and companies involved in the wind turbine-environment nexus with communities.
Penn State can attain leadership in wind energy with a modest investment, such as:
- Creating and supporting a center on wind energy.
- Adding additional faculty with proven research funding.
- Providing additional support for research, outreach, and educational activities.
Outreach efforts, such as the undergraduate Department of Energy-sponsored Collegiate Wind Competition that Penn State has won four times and consistently places in the top three teams, can be incorporated into such a center. At the undergraduate and graduate levels, there are already established curricula, notably with a certificate in wind energy offered through the College of Engineering. Greater coordination of the wind community at Penn State could quickly lead to a high visibility and greater impact in research and education for this large and diverse group.
Biomass
Penn State has established a Biomass Energy Center that was built on its success in being a leader in agriculture and many types of biomass production. The energy research at this center is focused on feedstock production and conversion, systems sustainability, carbon capture, and many aspects of the science surrounding lignocellulose, the main component of plant biomass. Researchers are part of the USDA Northeast Regional Sun Grant Center, and as well as other research teams with a focus on industrial biotechnology and the structure of lignocellulosic materials. Energy specific research spans fundamental studies on improving the biodegradability of biomass through engineering plants with more easily degradable walls or plants that grow faster, to issues associated with growing, harvesting, and sustainable growth of crops by focusing on nutrient recovery, biomass transport, and sustainability modeling. Because photosynthesis concentrates atmospheric CO2 in energy dense forms with diverse materials properties, biomass energy and materials systems can provide a large, near-term approach for achieving a negative carbon economy. Already carbon-smart bioenergy systems are sequestering CO2 in soil, bioproducts, and geological reservoirs at more than a megaton scale, but that needs to increase to 10 gigatons or more annually by 2100 to drawdown the projected overshoot of atmospheric CO2 concentration. There is a desire to invest in activities that will enable cross-cutting research in the economics, policy, law, business, and education needed for these carbon negative systems to rapidly expand. There are already excellent educational opportunities through courses offered in bioenergy, as well as a graduate program in BioRenewable Systems.
Thermal Systems
Current electrical power systems mostly rely upon thermal energy through conversion of fuels, for example natural gas to steam in turbines, and therefore there is extensive experience at Penn State in combustion, turbines, and heat transfer. However, there are tremendous opportunities for these scientists and engineers in geothermal energy, heat energy storage systems, and conversion of low grade (waste heat) into electricity. Worldwide, there is nearly 13 GW of geothermal power production, which is expected to grow by over 35% by 2020.
The research and activities in thermal processes related to geothermal energy by faculty at Penn State are generally not well recognized. However, Penn State researchers have expertise in simulations related to geothermal systems, and studies related to drilling, extraction technologies, and seismicity issues associated with fracturing processes. Areas of opportunity in thermal systems that have not been sufficiently exploited are in areas related to waste heat recovery, thermal batteries, and storage systems. Waste heat is a byproduct of many industries that could be a valuable resource if it could be more efficiently and economically converted to electricity. Typically waste heat sources are at temperatures below 230 oC, but additional opportunities exist even at temperatures below 100 oC. Strategic investment into thermal energy systems for waste heat recovery could generate research at Penn State that could quickly grow due to existing expertise in the fundamentals of thermal systems. Such areas could include thermal transport in batteries, nanomaterials for solid state thermal scavenging, reducing the cost materials (especially ion exchange membranes), and thermal control systems. Fostering greater interactions between thermal scientists, electrochemists, and materials researchers will be particularly important in building these new technologies.
Blue Energy
Blue energy, or the use of water with different hydrostatic or entropic energy differences, has for many years been a large percentage of renewable power generation. Conventional hydropower from dams contributes about 44% of renewable electricity in the US, and ~70% globally. Despite the seeming maturity and full penetration of hydropower systems to existing possible sources, there remain many new opportunities to capture energy from water sources as well as improve our existing (and sometimes aged) infrastructure.
There are thousands of dams operated in the US solely for flood control. Federal laws currently do not allow these dams to be used for both flood control and electricity production. However, such dual use could enable new, highly economical, and distributed sources of electrical power from our existing river system and flood control network.
Reservoirs could also function both as a dual method of water supply and energy storage. For example, it has been proposed to use the Hoover Dam as an energy storage system by returning water from the river back into the lake produced with the dam by using solar power.
There are other opportunities related to blue energy that include improving the cost and overall efficiency of water turbines by reducing operational and maintenance costs, and simple standardization of international designs. Other methods can be developed to extract energy from water-based systems, including novel methods to harvest tidal energy or advanced in situ river turbines. Electrical power can also be harvested from entropic energy, i.e. energy released when less-salty river water (or treated used waters) flows into seawater. Such salinity gradient energy could contribute globally as much as 1,000 GW of power if it could be efficiently and safely harvested.
The community working in blue energy at Penn State currently has no organized group. Moving more substantially into this area of research will first require a greater assessment of existing capabilities, as well as a planning effort in order for meaningful progress to be made in this field.
New Energy Ideas
Funding new ideas and approaches in renewable energy research is critical to enabling breakthroughs in energy conversion. Sometimes these ideas evolve from other venues of research. For example, research on salinity gradient energy showed that two streams having a difference in concentrations of chemical species, and therefore chemical potentials, could be an avenue for new power sources. This led to a new approach to capture energy from high concentrations of chemicals such as CO2 in flue gases through devices that mix this chemical with solutions lacking this chemical.
Interest in how energy can be derived from mechanical motion, such as expanding or contracting materials due to temperature or even humidity changes in the air could lead to new directions of energy research.
For all the above technologies mentioned in this report, new discoveries and approaches while working in even relatively mature fields could unlock new directions in energy research.
For example, a new method of electricity production was recently proposed by combining osmotic and heat energies to produce pressurized streams (an approach known as osmotic thermal batteries). Fundamental discoveries and innovation must both be encouraged to advance both technically feasible and economical harvesting of renewable energy for electricity production and other forms of fuels or chemicals.
Energy Storage and Use
Energy storage is an ever increasingly important component of transportation, housing, and communications and information. Large-scale energy storage is also critical to the renewable energy production infrastructure due to the intermittent harvesting of energy from some sources (such as solar and wind), but it is increasingly needed also to accommodate peaks and valleys in energy consumption to stabilize electrical transmission and the grid.
Batteries
Researchers at Penn State gained an early lead in research and development of electric vehicle (EV) batteries, and at one time led the nation in terms of funding and impact for their work. Currently, battery researchers at Penn State are organized through the Battery and Energy Storage Technology (BEST) Center, and funding levels for these faculty remains strong.
While the vitality and impact of this group is excellent, national leadership has been overtaken by other research teams in part due to greater strategic investment by other universities in this area. It is therefore important to help maintain the stability of the existing researchers through research infrastructure investment, for example, through dedicated space for battery construction and pouch cell fabrication, and other electrical testing equipment. Expansion of the center into larger-scale energy storage, or novel approaches based on integration of battery-powered vehicles into the grid (for charging the vehicles, or using charged vehicles as energy storage devices), will require additional investment into faculty hires in the area of grid storage and power electronics.
It is also notable that research in stationary flow batteries, an area of active research for energy storage, is nearly absent at Penn State. While a conventional battery is a complete structure, flow batteries are based on storing energy in liquid electrolytes in large tanks, with the solutions pumped through the battery to create a discharge cycle disproportionate to that of the size of the battery itself. Further investments in batteries are an essential component of both renewable and other energy technologies for the future.
Hydrogen
Although much attention is focused on hydrogen relative to fuel cells used to power automobiles, hydrogen is currently much more in demand in the US for fertilizer production via the Haber-Bosch process that produces ammonia. Hydrogen is also extensively consumed for gasoline and methanol production, and used as a rocket fuel, among many other industrial and consumer products.
Most hydrogen today is produced from fossil fuels, directly linking its use to CO2 release into the atmosphere. The National Renewable Energy Laboratory (NREL) is focusing much of its activities on solar hydrogen production, with an emphasis on a two-stage, solar-to-electricity, and electricity-to-hydrogen by water electrolysis.
Penn State has a Hydrogen Energy Center which has historically been focused on hydrogen production from renewable biomass sources, fuel cell research and development, and fuel cell vehicles. Penn State also hosts a hydrogen refueling station, although it is currently inactive due to a lack of sufficient funding for vehicle demonstration programs outside of California.
Hydrogen and fuel cell research and applications remain a vital part of our energy infrastructure, as hydrogen is an excellent method for energy storage, and fuel cell vehicles typically have much larger driving ranges before being refilled compared to electric vehicles.
However, given the relatively low and very competitive funding available in the US, Penn State would make greater relative gains going into the future by investing more generally in research in electrochemistry and electrochemical hydrogen production, rather than fuel cell vehicles or transportation projects. Water electrolyzers and hydrogen evolution catalysts are critical components to hydrogen production and use in our energy infrastructure. Linking solar and hydrogen researchers could stimulate innovation in the energy sector as well.
Pumped Storage
Pumped water storage to high-elevation reservoirs remains the single largest method for stored energy in the world due to the relatively high efficiencies of water turbines and pumps. Pumped air storage into caverns is also a viable method for energy storage, although it is less widely applied due to the need for existing caverns to store the pressurized gas. Because of the reliance of these systems on turbines, researchers working on improving turbine operational duration, and reduced maintenance and costs, could also interface into pumped storage energy systems. Thus, investments in energy production from water systems could similarly benefit advancing applications of storing energy in elevated water reservoirs.
Interdisciplinary Communities
Electrochemistry
A recurring component in almost all of the energy systems is the reliance on fundamental aspects of electrochemistry, as well as innovations in applied electrochemical systems. Electrochemistry and catalysis are fundamental aspects of electrochemical water splitting (which produces oxygen or chlorine) and the hydrogen evolution reaction, current generation in solar cells, chemical production using CO2, and electricity production using fuel cells.
Electrochemistry not only has a central role in batteries, it is also important to many fundamental scientific topics such as surface chemistry, capacitors, and ion transport. The importance of electrochemistry was highlighted by the recent creation of an “Electrochemical Systems” program in the Division of Chemical, Bioengineering, Environmental, and Transport (CBET), in the National Science Foundation (NSF).
There are several researchers at Penn State with a reliance on fundamental electrochemistry principles, with notable classes in the College of Earth and Mineral Science that support an undergraduate minor and a graduate minor in electrochemistry topics. There is also research into chemical separation technologies, and new applications such as electrochemical desalination.
There are a few universities that have leadership in electrochemical processes, but currently Penn State is not among them. However, with some organization and better visibility of the work already being done at Penn State, and some additional strategic investments in faculty and research, Penn State could emerge into this role as a leader in this area, particularly with the high number of engineers graduating from the University who will be integrated into an increasingly electricity-dependent society. What is first needed for electrochemistry research and education is an evaluation of the best way to proceed in building this area to greater national prominence.
Membranes
Many different types of energy systems use membranes, for example batteries where they can function primarily as separators between electrodes, fuel cells where ion selective transport and stability at high temperatures is important, water electrolyzers which are used to produce hydrogen gas, and different chemical separation processes. The properties and the cost of the membranes central to the economic competitiveness of existing and emerging technologies as the membranes directly impact their cost and lifetimes. Researchers who develop membranes are often different than those who use them in different applications, and therefore it is important to create a cohesive membrane community at Penn State.
A new integrative center funded by the National Science Foundation, through the Industry–University Cooperative Research Centers Program (I/UCRC), can take a leadership role in raising the visibility of membrane-based research at Penn State by helping to coordinate strategic hires in membrane research and applications, increasing research funding in these areas, and promoting the development of courses and other educational activities focused on membranes.
Education
There are a large number of classes, undergraduate and graduate minors, and certificate programs, and world campus classes and degrees related to sustainable energy already in existence at Penn State, although their collection into an easily navigable website or location does not yet exist. It is difficult to make specific recommendations on educational priorities at this point due to a lack of collected information. However, the strategic reports on the individual areas highlighted in this report (which can be found on energy2100.psu.edu) list many courses and educational programs with relevance to renewable energy, and these lists are a good first step in forming a more defined connection of these different educational efforts in renewable energy.
A new task force should be assembled and charged with the goal of collecting these courses into a cohesive website, hosted within the Institutes of Energy and the Environment website, to better frame the large community that exists in renewable energy research in terms of educational opportunities. This task force could also be charged with assessment of the extent of incorporation of renewable energy technologies, and especially electrochemistry and electrochemical engineering content, into the undergraduate curriculum.
Assessment, Community Involvement, and Outreach
Assessment of new technologies is an important component for successfully moving new technologies into our energy systems. Reworking our current fossil-fuel centric energy economy into a flexible, renewable energy-focused infrastructure will require many changes in technologies, lifestyles, and our infrastructure.
Before undertaking changes that impact our local and global communities, we need to carefully assess possible impacts of these changes and anticipate or survey community responses. One approach is to first consider how to assess the sustainability of the changes being proposed. This can be accomplished through research and modeling of the integrated impacts of the physical, socioeconomic, and policy changes and their outcomes relative to different technical choices.
There are researchers at Penn State focused with capabilities in these various assessment tools, which include approaches such as life cycle analysis, supply chain analysis, and modeling the impacts of decision-making under uncertainty. A center on Sustainability Assessment and Modeling could synthesize these different approaches for cohesive research training, and education through new courses and training programs, with a near-term focus on renewable energy technologies. There is particular interest in this area in Chemical Engineering and Agricultural and Biological Engineering, although there may be many opportunities in other departments to expand efforts in this area.
Social scientists can play important roles in effecting changes in our energy infrastructure. University professors are used to disseminating information through classes, where students have signed up to learn about a certain topic. When people are actively engaged in learning in the classroom or through outreach activities, it is easier to assess responses to proposed changes in this controlled environment than in a community. Outside the classroom, it is important to understand that opinions on energy technologies may already be set for a large proportion of a community based on socially shared information or information obtained through personal experience. Such generally acquired wisdom can greatly influence how communities will respond to changes, as it can outweigh the facts laid out in a classroom setting that describe the benefits of changes to communities. Technology development and translation into a community, and deployment of an effective workforce to advance these new technologies, cannot effectively proceed without sufficient understanding of the responses of communities to changes in our energy lifestyles.
Social science methodologies can be used to better understand how people discuss and view these new energy technologies, and identify potential barriers or bottlenecks for change. A series of workshops and seminars could start to bridge the gaps between basic, applied, and social science researchers at Penn State, as a method of better preparing these groups for collaborating on interdisciplinary center proposals. Funding for additional faculty with expertise in psychology, sociology, decision science, and science communication will benefit the transformation of the country’s energy infrastructure to a more sustainable system.
There are existing and targeted outreach activities related to the energy, water, and food nexus, with several of these activities fitting well within the topic of renewable energy. Some examples are: the PA Wind for Schools program (funded by DOE), an annual PA KidWind Competition that is held on the University Park campus, Teacher Professional Development Workshops that are offered several times each year on wind energy, Center for Science in the Schools teacher training programs on Carbon Earth and Biomass Energy, and energy extension programs on Energy 2100 topics including biomass energy and solar.
One challenge in these efforts is maintaining stable funding for these outreach activities. It is suggested that these activities be more comprehensively assessed, and leadership be established in overseeing and coordinating these activities into more sustained programs over time. For example, the PA Wind for Schools program is working on a fundraising campaign to continue work in this area, with the new program called the PA REpowering Schools. And there is an ongoing discussion of how to organize and deliver energy extension programming in a more coordinated way.
Linking Groups Together
One overall aim of this Strategic Initiative is to link groups together for a more integrative assessment of how renewable energy technologies can be synergistically combined. For example, as noted above, large-scale adoption of intermittent technologies for electricity generation, such as wind and solar, requires integration with grid and storage technologies. Planning for the deployment of new energy systems requires consideration of technical feasibility, environmental drawbacks and benefits, economic viability, social acceptance or resistance, compatibility with legal and policy frameworks, and time horizons. New approaches in bringing people together across the campus, rewarding collaborations, and stimulating new research directions will require thoughtful and creative planning by many groups composed of individuals with diverse backgrounds.
Outcome Assessment
Energy 2100 is a strategic initiative, with funding and goals to invigorate research and activities in the renewable energy portfolio at Penn State. Many other activities will also be occurring as a result of strategic decisions within departments, for example hiring new faculty or offering new courses, that should be assessed within the time-frame of the initiative. Therefore, it is recommended that within 2 years from the issuance of this report, Penn State should convene a panel of experts to review our renewable energy portfolio, activities, and plans, and evaluate the success of these activities as well as make further recommendations for future activities. Such an external review can aid in identifying ways to further the impact of our activities within the context of renewable energy.
Conclusions
Penn State is a leader in energy research, and it has many new opportunities to achieve greater international prominence in renewable energy research, education, and outreach. In many instances identified in this report, small strategic investments can catalyze large and impactful advances in renewable energy technologies. The most critical steps in many of these technical areas involve bringing communities of researchers together in the form of working groups or centers, providing seed funds to draw existing faculty into new research directions related to renewable energy, making targeted investments in faculty positions in key areas, and supporting the infrastructure needed by groups of researchers in certain areas.
There are certain areas, for example solar energy research, where the existing group is entering a very fragile existence, and immediate attention is warranted to replace departing faculty and to bring in new faculty to stabilize the community and energize this direction of research. In other instances, the short-term goal is to link groups that focus on specific topics to better integrate with others outside of their usual research communities.
Key to the success of all of these activities is long-term investment through gifts and endowments to key faculty positions and research topics and the support of faculty that are driving change in renewable energy research.
Penn State should continue to enhance its efforts to share information with the community, its alumni, and others, regarding the tremendous success and future potential of the researchers working in the development of new, beneficial energy conversion and storage technologies.